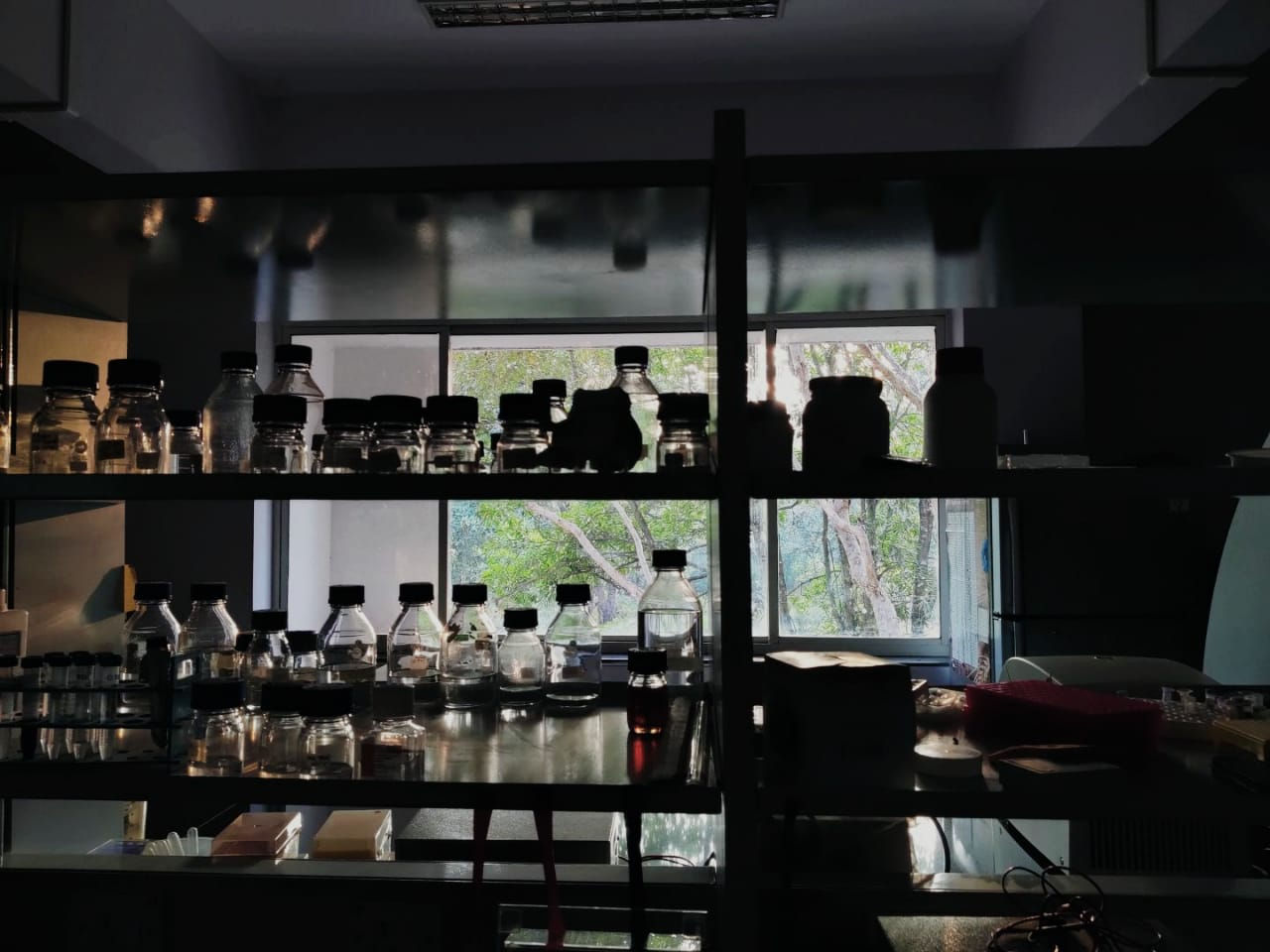
Peptidomimetics Laboratory @JNCASR
1. Understanding various aspects of carbonyl-carbonyl n→π* noncovalent interaction
(This project is supported by DST-SERB for Early Career Research Award Grant 2016-2019)
Carbonyl-carbonyl n→π* interaction has emerged as a novel noncovalent interaction that not only influences the geometries of small molecules such as aspirin and homoserine lactones but also plays important roles in determining the three-dimensional structures of peptides, peptoids, and proteins. In carbonyl-carbonyl n→π* interaction, one of the lone pairs on the oxygen atom of a carbonyl group is delocalized over the antibonding π* orbital of a nearby carbonyl C=O bond. It is characterized by the Burgi-Dunitz trajectory of the approach of donor oxygen to the electrophilic carbonyl carbon acceptor atom. It is now well established that short O•••C=O distance (d) of less than 3.22 Å (the sum of van der Waals radii of carbon and oxygen atom is 3.22 Å), bond angle ∠O•••C=O (θ) of 109 ± 10°, and the pyramidality (Δ) of the acceptor carbon atom are the primary characteristics of an n→π* interaction. The role of carbonyl-carbonyl n→π* interaction on molecular structure and properties is now well studied. However, there are many unanswered questions regarding this interaction. We are trying to understand various aspects of CO•••CO n→π* interaction through design, synthesis, and systematic studies of suitable model compounds.
2. Understanding the role of n→π* interactions in the conformational properties of N,N'-diacylhydrazines and azapeptide models
(This project is supported by DST-SERB for Core Research Grant 2020-2023)
A delicate balance of steric and electronic factors determines the minimum energy conformation of molecules. In recent years, n → π* interactions between the lone pair of a donor atom and antibonding π orbital of a nearby acceptor π-system has attracted considerable attention. It is now well known that two nearby carbonyl groups can involve in attractive interaction via n → π* electron delocation where the oxygen atom of one carbonyl group donates electrons to the π* orbital of the C=O bond of the other carbonyl group. Our recent studies indicated the presence of “reciprocal” carbonyl-carbonyl n → π* interactions between the neighbouring carbonyl groups of unsubstituted N,N'-diacylhydrazines. Our ongoing investigations suggest that N-methylation of diacylhydrazines drastically changes their geometry due to cis-trans isomerization of the amide bonds and n → π* interactions play a crucial role in their conformational stabilization. This is important as RH-5849, a 1,2-diacyl-1-tert-butylhydrazine that mimics ecdysone, completely loses its insecticidal activity upon N-methylation. This could be due to loss of hydrogen bonding interaction in the active site of ecdysone receptor upon N-methylation or because of loss of binding affinity due change in the amide bond geometry post N-methylation. Therefore, it is important to understand the role of substituents on the geometries of an N,N'-diacylhydrazine to improve our understanding of N,N'-diacylhydrazine-based drug development. Earlier studies on the conformational properties of diacylhydrazines and other analogous aza-containing molecules including monoacylhydrazine and azapeptides overlooked the importance of n → π* interactions in determining their conformational stability. Our ongoing investigation suggests that such n → π* interactions can have dominant role in determining the minimum energy conformation of these molecules. Understanding the role of noncovalent interactions in diacylhydrazine and azapeptide model compounds could shed light into the geometries of various aza-oligomeric structures.
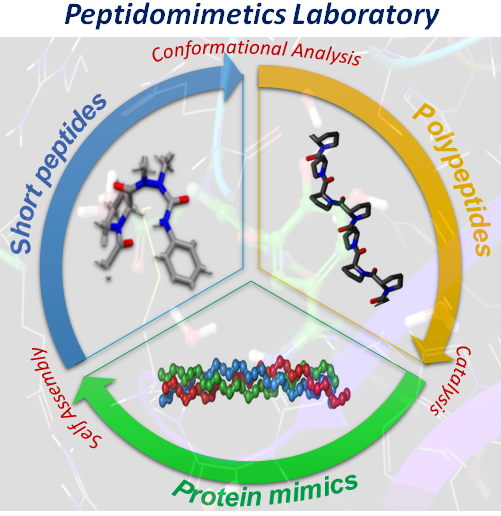
3. Understanding the stabilities of polyproline helices
The tertiary amide bond of proline (Pro) can adopt cis conformation more readily than the secondary amide bonds of other naturally occurring amino acids. Because of the facile cis-trans isomerization of the Pro amide bond, poly-Pro (poly-P) tracks can adopt either polyproline I (PPI, all cis amide) or polyproline II (PPII, all trans amide) helical conformation in solution in a solvent-dependent manner. Poly-P often forms left-handed PPII helical geometry in aqueous and other polar solvents such as trifluoroethanol but prefer a more compact right-handed PPI helical geometry in less polar and hydrophobic solvents such as n-propanol and other aliphatic alcohols. Unfortunately, unlike the α-helices and β-sheets, the origin of stabilities of PPI and PPII helices are poorly understood. We are focused on getting molecular level understanding of the conformational properties of PP helices through the design and synthesis of short poly-P tracks.
4. Design of synthetic collagen mimics for biomedical applications
Collagen is the most abundant protein in animals and constitute ~70 percent of the dry weight of human skin. Abnormalities in collagen structures are associated with a wide variety of human diseases including arthritis. Collagen is useful in the regeneration of damaged tissue such as nerve, bone, skin, or vascular tissues. While natural collagen has many clinical advantages for such applications including biocompatibility, the use of human and animal-derived collagen presents potential hazards, especially the transmission of infectious agents from donor to donee. Therefore, simplified peptides or peptidomimetic structures having collagen-like properties for similar clinical applications present attractive alternatives. Our group is interested in strategies to design and synthesize hyperstable artificial collagen mimics and their self-assembled hierarchical structures such as nanofibers and hydrogels with potential biomedical applications.
5. Developing fluorescent probes to sense monoamine oxidases in the brain
Monoamine oxidase controls the level of monoamine neurotransmitters in the brain. However, abnormal activity of MAO can lead to significant depletion of the neurotransmitters as well as overproduction of H2O2, which are linked to depression and neurodegenerative diseases, respectively. MAO has two isoforms — MAO-A and MAO-B. Abnormal activities of MAO-A and MAO-B are linked to depression and Parkinson’s disease (PD), respectively. Therefore, continuous monitoring and maintaining an optimum level of MAO is necessary for the proper functioning of the brain. One of the major challenges in tackling brain diseases and disorders is the continuous detection and monitoring of the disease progression. This is largely due to the lack of blood-brain-barrier (BBB) permeable chemical probes to monitor the events linked to these diseases. Fluorescence-based methods are suitable for these purposes as they are highly sensitive and amenable to deep-tissue bioimaging. The existing fluorescent probes of MAO have many limitations. The irreversible inhibitor-based fluorescent probes often possess poor blood-brain-barrier permeability and poor signal-to-noise ratio. On the other hand, in case of the substrate-based fluorescent probes, the fluorescent molecule produced in situ after the metabolism of the substrate may diffuse away from the enzyme pocket, which makes localization and monitoring of MAO challenging. We are trying to develop fluoroscent probes that can overcome these problems.